Highlights
- The role of carbon capture, utilization, and storage technologies (CCUS) is a contentious part of the clean energy transition debate. While some argue that the shift towards net zero can be achieved without their use and focus here may divert much needed capital and attention on scaling other clean technologies, there are important reasons for why carbon capture should be included in the toolkit in the fight against climate change.
- CCUS plays a significant role in emissions reductions in most net zero scenarios used today, including those by the Intergovernmental Panel on Climate Change and the International Energy Agency, which recognize that despite best efforts to full decarbonization strategies, there may still be residual emissions for which carbon capture represents part of the solution for reaching that final mile. These include areas like hard-to-abate sectors, fossil fuel byproducts for which there are limited sustainable alternatives, and in supporting the development of other clean technologies like biofuels.
- Canada has a significant opportunity to establish itself as a leader in CCUS as it is one of only a few countries that have made significant inroads in developing and using this technology. A strong dependence on high-emitting sectors that may make use of it, along with the potential growth opportunity in the clean energy transition provide strong reasons for policymakers to consider supporting its development.
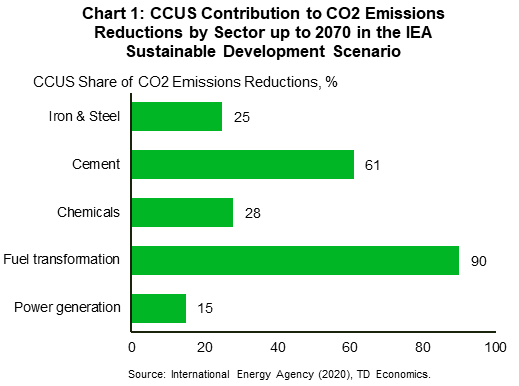
Carbon capture, utilization and storage (CCUS) technologies and their role in the clean energy transition is a highly contentious issue in the climate debate. Some negatively view this investment as a means to maintain fossil fuel production with the goal to delay or avoid efforts to adopt clean technologies and infrastructures on the path towards net zero. However, there are two important perspectives for why CCUS will be needed to succeed in meeting net zero goals while limiting energy insecurity. First, the world faces a significant challenge in rapidly decreasing its dependence on fossil fuels in the timeframe needed to reach the interim goals in the Paris climate accords. Hard-to-abate sectors, fossil fuel byproducts for which there are limited sustainable alternatives, limited long-duration energy storage solutions, and challenging behavioural shifts create difficulties in migrating to a 100% renewables-based energy system. Second, there are important net zero technologies, such as clean fuels, whose viability is dependent on the same carbon capture technology and infrastructure that might be used in the fossil fuels sector, including bioenergy and clean fuels. It is for these reasons that CCUS will likely play a critical role in the transition to net zero, both as an insurance policy, in case behavioural shifts are either not broad enough or fast enough, and as a transition technology to help scale clean energy technologies that will be needed. Lastly, timing is a serious issue given the speed with which emissions need to come off to limit global warming to 1.5°C – every possible technology that can help reduce emissions thus needs to be considered.
For those unfamiliar with CCUS, it is a process of removing carbon dioxide emissions from the air or emitting sources and either storing it underground or using it in ways that it does not emit back into the atmosphere. Capturing CO2 relies either on technology built directly into production processes (point-source capture), or removal from ambient air using nature-based CO2 sinks or direct air capture. And capturing the emissions is only a small piece of the puzzle. It then requires processing, transporting, and permanent storage or reutilization. Note in this report, we align with the IEA and the Canadian federal government in using CCUS as the umbrella term for all natural and technological methods of capturing, utilizing and storing emissions, whether from an individual source or from the ambient air. Capturing CO2 is not an easy undertaking and requires considerable planning. Each part of the CCUS chain requires capital and technological investment and, in some cases, further scientific advancement. But, it is worth noting that most widely held views about the clean energy transition incorporate CCUS in some way. Recent emissions reduction scenarios generated by inter-governmental agencies such as the International Energy Agency (IEA) and the UN Intergovernmental Panel on Climate Change (IPCC) all rely on varying levels of carbon capture implementation to achieve net zero emissions by 2050. The IEA, for example, estimates the total price tag for global annual investment in the CCUS space to exceed USD $160 billion by 20501, acknowledging that despite best efforts to commit to full decarbonization strategies, there may still be residual carbon emitted into the air. To that end, carbon capture is part of the solution for reaching that final mile.
It is here where Canada's opportunity lies. Canada is one of just a few countries that have made serious inroads in both developing and using carbon capture technology along with building infrastructure to transport and store its captured emissions. In the many ways in which this technology might be used, including in biofuels, hydrogen and as a standalone industry, Canada stands apart.
The Case for Carbon Capture in the Clean Energy Transition
The contentious issue of CCUS results in many different perspectives. Those against it advocate that the shift to net zero is possible through the exclusive use of renewable energy sources, eliminating any need to invest in either continued fossil fuel production or CCUS. This pathway eliminates concerns about the efficacy and viability of CCUS, or that investments in CCUS might divert needed capital from developing and scaling cleaner energy sources and technologies. The continued usage of fossil fuels also suggests a continued reliance on fossil fuel byproducts, which contribute to broader environmental degradation, such as the impact of plastic waste on a variety of ecosystems. Importantly, many advocates against the use of carbon capture claim that investing in these tools delays and avoids the necessary shift away from fossil fuels within our energy system needed to combat climate change
There are several challenges in completely replacing fossil fuels with renewables, while also ensuring the reliability of the energy supply over the course of the transition. Technical difficulties arise in decarbonizing hard-to-abate sectors such as fertilizers, aluminum, steel, cement, petrochemicals, etc, for clean fuels production, such as for biofuels, for firm/dispatchable electricity generation.
In this regard, investing and scaling CCUS technologies is akin to an insurance policy. In an ideal world, the best possible effort is made to reduce our dependence on fossil fuels, massively scale up the production of renewable energy and decarbonize as many end-use services as possible. However, even in that ideal world, there may still be activities for which decarbonization is not viable. In those situations, CCUS represents that final line of defense to ensuring there is a way to capture those last emissions.
Finally, there's the notion that CCUS technology plays a broader role in the clean energy transition than just capturing emissions in fossil fuel production and other heavy industries. Critical components of most net zero scenarios require the production of bioenergy, clean fuels, and carbon dioxide removal (CDR) technologies. It is CCUS technology and infrastructure that underpins their viability. In a 2019 report, the Intergovernmental Panel on Climate Change acknowledges that, "All analyzed pathways limiting warming to 1.5°C with no or limited overshoot use CDR to some extent to neutralize emissions from sources for which no mitigation measures have been identified and, in most cases, also to achieve net negative emissions to return global warming to 1.5°C following a peak."2 The IEA Net-Zero Emissions by 2050 Scenario (NZE) released in 2021 details the necessary steps and available routes to decarbonization using existing and anticipated technologies. In this scenario, even after all renewable energy sources have been adopted and scaled, and all existing decarbonization shifts have been completed, they estimate that 7.6 gT of CO2 per year will still need to be captured. Global GHG emissions currently sit at approximately 47.6 gT of CO2-equivalent per year, giving some sense of scale of how much carbon needs to be sequestered annually.
For a country like Canada that has a high dependence on fossil fuels both for its energy system and economy, CCUS is likely to play a disproportionate role in the clean energy transition by serving multiple functions. Fossil fuels play a large role directly in Canadian GDP and employment, as well as the spillover effects on incomes, innovation and competitiveness. A hasty transition to renewables without deliberation for what workers and households will transition to is likely to threaten both economic prosperity and potentially the reliability of our energy supply. If this outcome were to occur, it would not just undermine future efforts towards net zero, it could throw the gear into reverse as evidenced in other parts of the world. On the same token, there is a responsibility among Canadian producers given their high carbon intensity of production to show global leadership in this space by leveraging their capabilities in project management and innovation and showcase how sustainable production can be a crucial tool in the fight against climate change.
Where will CCUS be used?
CCUS technology should not be used to avoid or substitute for decarbonization where other technological solutions exist that can be appropriately scaled and applied, but rather as a solution for hard-to-abate sectors that have limited decarbonizing pathways based on their specific industry demands or procedures. CCUS is a particularly important piece of the clean energy transition plan where hydrogen becomes a dominant energy source for application in the transport, industry and building sectors. Collectively, these sectors contributed to 56% of total global energy-related CO2 emissions in 2018.3 For one heavy industry, cement production, CCUS is one of the only currently known technology solutions for considerable emissions reductions.4 Overall, CCUS has the potential to account for between 15% and 90% of emissions reductions in the iron and steel, cement, chemicals, fuel transformation and power generation sectors by 2070 (Chart 1).
In addition, fossil fuels may still need to exist in the overall energy profile of 2050. By then, the IEA estimates 120 EJ of fossil fuels will be consumed for a few applications: non-combusted input use, reduction of stranded assets and preservation of the lifetimes of young facilities, and to support hard to abate sector operations which are projected to still emit 1.7 Gt CO2/year emissions in 2050. To the extent these fossil fuel use practices generate CO2 emissions, CCUS technologies are needed to ensure the removal of CO2 emissions from the atmosphere on a net basis.
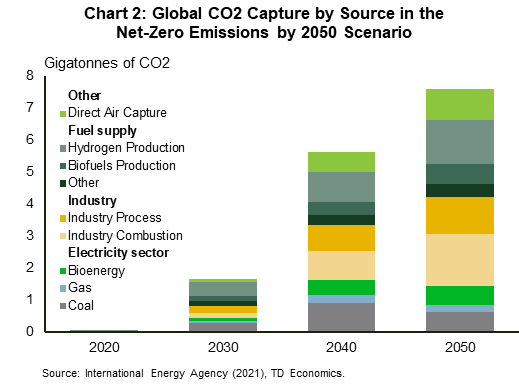
For many of these energy-related emitting sectors, capturing CO2 directly at the facility, called point-source capture, is an efficient CCUS technology solution. CO2 concentrations at production site processing are significantly greater than directly from ambient air, which is the alternative CCUS method called carbon dioxide removal (CDR). The estimated concentration of CO2 in the atmosphere is roughly 0.04%, compared to ~4% in flue gas from natural gas-fired plants and 12-15% from coal-fired plants.5,6 This underpins the NZE assumption that 69% of the captured 7.6 Gt CO2/year will be from point-source capture technologies across a variety of applications (Chart 2).
The remaining 32% of captured CO2 by 2050 is expected to come from CDR methods. CDR is an option for those industries that cannot fit point-source capture technology into their processes. This would be the best solution for firms whose emissions do not occur through a facility processing, but instead through their operation, such as through critical inputs or consumption of materials. One example is the aviation industry, where CO2 emissions during fuel consumption cannot be captured using point-source technology. To this end, CDR methods can be employed to support a clean energy solution for long-haul transportation, such as "blue hydrogen", an innovative clean fuel alternative. Blue hydrogen is produced from natural gas or coal and the resulting emissions are captured using a CDR method (discussed below), which is the lowest cost option to produce hydrogen relative to renewables-based generation.
Finally, CDR methods can also be used by companies who are not part of the energy-emitting sectors but still need to reduce their overall emissions profiles in achieving net-zero for their organization. For example, Microsoft announced in January 2021 that it has used CDR methods to remove 1.3 Mt CO2 from the atmosphere, and looking ahead, has secured a contract with direct air capture company, Climeworks, to remove 11% of its total annual emissions.7
CCUS Basics
Point Source Capture
In point-source CCUS, carbon capture technology is implemented into a facility's production process. There are four basic systems for point-source capture: industrial process streams, post-combustion capture, pre-combustion capture, and oxy-fuel capture.
Capture from industrial process streams is an existing technology employed by industries for decades, where CO2 capture is embedded into the original processing cycle for a specific purpose. Historically, captured emissions were released absent incentives to store it. Another process like post-combustion, pre-combustion, or oxy-fuel capture is used in combination to complete the capture cycle. Examples of industrial use include purification of natural gas, production of hydrogen-containing synthesis gas for the manufacture of ammonia, alcohols and synthetic liquid fuels, and cement and steel production.8
Post-combustion capture is the process of capturing the CO2 emissions from flue gas after the combustion of a fuel source. It is most notably used in oil, coal and natural gas power facilities. The CO2 in the post-combustion capture process is emitted alongside many other substances in the gas stream, requiring further processing and compression. By comparison, pre-combustion capture is more efficient in CO2 capture as it is extracted before the combustion process, allowing for purer carbon to be captured. Finally, oxy-fuel capture occurs when fuel is burned in a pure oxygen chamber instead of air, resulting in the purest stream of CO2 of all the processes.
Carbon Dioxide Removal
When point-source capture is not a viable solution, the other option is to remove carbon dioxide directly from the ambient air using carbon dioxide removal (CDR) strategies. This can be done using nature-based carbon sinks or through large technology systems called Direct Air Capture (DAC).
Nature-based CDR methods rely on natural carbon sinks that extract CO2 from the air and absorb it into the earth. Methods include afforestation/reforestation, biochar, enhanced weathering, ocean fertilization and bioenergy with carbon capture and storage (BECCS). Afforestation/reforestation and BECCS are the most explored nature-based solutions to date. Figure 1 broadly outlines the CO2 removal processes through these methods.
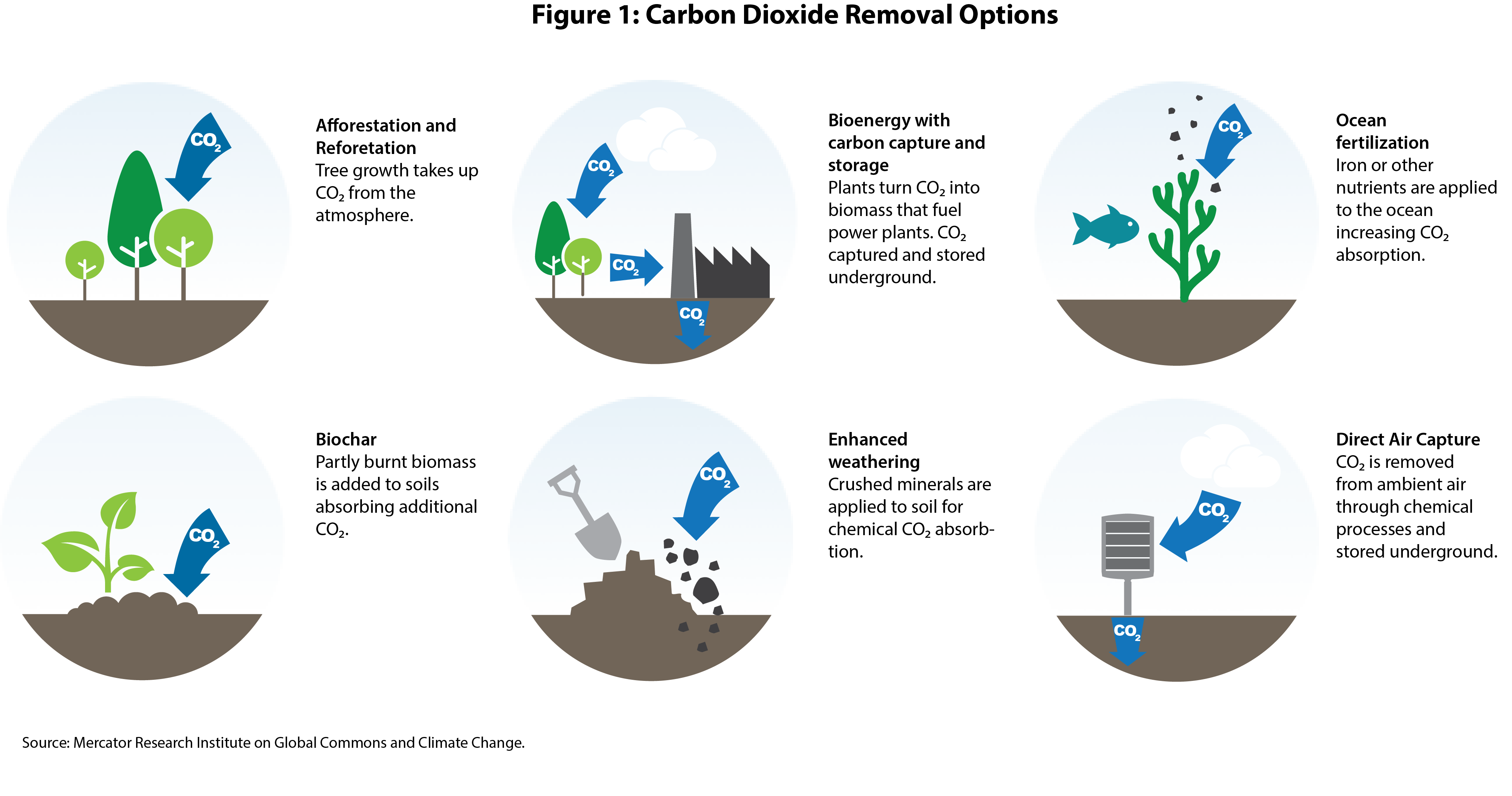
Barriers That Lie Ahead
The current carbon capture technology landscape provides opportunities for companies to customize their approach to best suit their production needs. Each method has its own advantages in its operation and use, but alongside that comes barriers to overcome.
Point-Source Capture: High cost, potentially high reward
Digging deeper into point-source capture reveals capital cost barriers that companies will need to consider. These costs are broken down into two balancing segments: fixed cost of the initial technology and variable cost for the processing and purification of CO2 from other emissions. Dilute gas streams face higher cost as the process of isolating carbon dioxide from the other emissions requires more effort and technology compared to purer streams.
Post-combustion capture is the lowest fixed cost opportunity as the capture technology can often be retrofitted into existing facility sites and the technology is well understood. However, the gas stream produced is the most dilute and requires a higher variable cost to extract the CO2. By comparison, pre-combustion capture releases purer streams of CO2 thus requiring less variable processing costs, but upfront capital investment costs for developing pre-combustion capture technologies may be higher than comparable post-combustion solutions.9 Finally, oxy-fuel capture releases an emission stream of almost pure CO2, making it the simplest to process. However, the technical and capital input requirements of oxy-fuel capture limit implementation, as majority of the oxy-fuel sites are still in testing and pilot stages.10
Fixed cost estimates for the capture technology vary based on the facility status and technology chosen. Once the technology is in place, the IEA estimates costs for processes producing highly concentrated CO2 streams, such as ethanol production or natural gas processing, between USD $15-25/t CO2, while processes with "dilute" gas streams, such as cement production and power generation, face cost estimates between USD $40-120/t CO2.11
This is all to say that a company exploring point-source capture will first need to assess their current operation framework to determine which technology system best suits their needs, and whether it can be implemented into existing sites. Retrofitting is one cost-saving strategy but may not be an option for all processing plants. That means that new facility development that introduce carbon capture technologies may need to be incorporated in a businesses' long-term planning.
CDR: Capture flexibility, but with large claims to resources
However, there are some notable challenges with CDR. As the concentration of CO2 in ambient air is far less than directly from the source, companies will have to scale CDR technologies drastically to meet their targets. For nature-based methods like afforestation/reforestation and BECCS, this will require a non-negligible claim on the world's land resources. Depending on the scale of CDR deployment, this may crowd out valuable land resources for other evolving global concerns like food production.
DAC, by comparison, has a smaller land requirement than nature-based solutions and site location does not claim arable land use. DAC technology accounts for 10% of total capture amounts in the NZE scenario, scaling from current low levels of pilot projects today to 90 Mt CO2/year in 2030 and just under 1 Gt CO2/year by 2050. However, there are some considerable limitations to DAC.
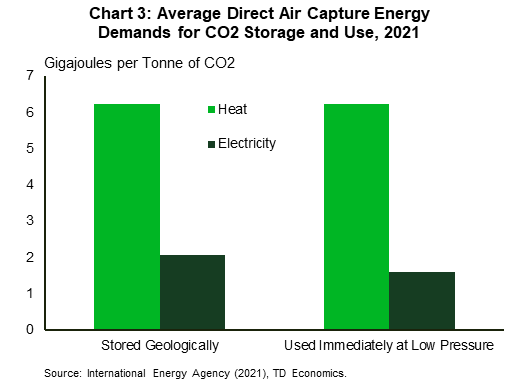
First, the technology is expensive. Costs for DAC vary between $250-$600/tonne of CO2 depending on the technology choice, low-carbon energy source and the scale of their deployment. For context, most reforestation costs less than $50/tonne.12 Second, it's energy intensive. Heat is required to extract CO2 from the capture sorbent, making heat energy the most expensive input cost for its output yield, measured in gigajoules per tonne of CO2 captured (Chart 3). To make this process carbon-neutral in the long run, DAC will benefit from finding renewable or clean energy input sources. In scaling DAC to become an impactful solution for carbon capture technology, companies will have to consider the financial and environmental tradeoffs between the currently low CO2 capture yield and the energy input it requires to operate.
While complex, the scaling of DAC is going to be an important element of the transition as it and afforestation will be important sources of emissions sequestration. This goes to the complexity of how emissions are generated. While renewables, clean technologies and behavioural shifts will account for the lion's share of emissions reductions on the path to net zero, there are instances in which emissions may still be generated. In many of those instances, for example in hard-to-abate industrial sectors or what fossil fuel production remains, point-source capture will act as a potential pathway in reducing those emissions. However, there may still be instances in which point-source capture is not either possible or that smaller sources of emissions fall under the radar in the myriad behavioural shifts that need to occur. The IEA estimates that CDR accounts for roughly 1.9 gT of CO2-equivalent will need to be captured by 2050 in their net zero scenario.
The final piece: Captured carbon needs to be moved to storage
Once the carbon emissions are captured, a big piece of the puzzle left is how to store it to remain out of the atmosphere and how to get it there.
Compressed CO2 emissions are transported via ships and underground pipelines. Existing pipeline infrastructure provides a good starting point for CO2 transport; however, more pipeline links are required to facilitate connections between all potential CO2 capture sites. Princeton University estimates that in the U.S., around 110,000 km of new CO2 pipeline infrastructure will need to be built with an estimated capital cost of $170 to $230 billion. Such large capital requirements may seem like a diversion of funds from other clean technologies that could benefit significantly from these same investment dollars, but without carbon capture, the net zero goal may prove elusive. And this likely only amounts to a very small fraction of what is ultimately needed to reach net zero when the full transition tab is added up.
Carbon is then stored deep underground in rock formations or empty oil reservoirs. There is ample available global storage space for anticipated capture levels of CO2. Empty oil reservoirs are a common and well-documented storage case for CO2 since the 1970's, thanks to an oil recovery practice called Enhanced Oil Recovery (EOR). In EOR, CO2 is pushed down into oil reservoirs to extract the third, tertiary, round of oil from the reservoir. The CO2 is then captured and stored in the empty reservoir. Dedicated geological storage sites are another option, where the carbon is permanently stored in impermeable rock formations in perpetuity. Finally, there are cases where CO2 can be reutilized into non-emitting business processes, like food and beverage or as an input to synthetic gas and liquids. However, the carbon needs in these reutilization instances cumulate to a relatively small share of overall storage demand.
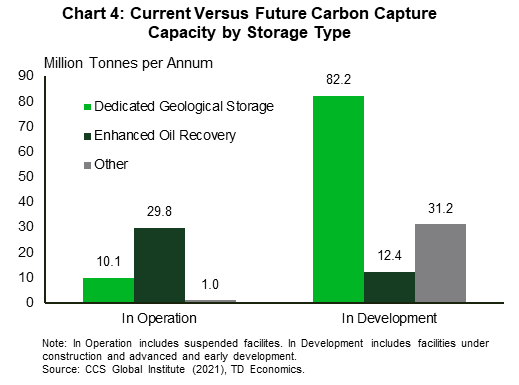
At present, 75% of current CCUS facilities in operation today send captured CO2 to EOR storage sites for oil and gas extraction. As oil and gas demands wanes in net-zero targeting scenarios, storage sites for future facilities in development are expected to favor dedicated geological storage. Looking ahead, 79% of all in-development sites plan to use dedicated geological storage (chart 4).
The transportation and storage piece of the carbon capture narrative is increasingly important when weighing CCUS technology options as businesses will be sensitive to distances between capture and storage sites. For example, if the point-source capture location is far away from an eligible storage site, either transportation will need to be arranged or another capture technology, like direct air capture that is less site-sensitive, will need to be explored. CCUS implementation will need to factor in transportation and storage solution at the onset of planning to ensure the feasibility of a company's overall capture strategy.
Canada's Opportunity
Technical challenges related to CCUS are many and form the basis of the argument that if such commitments to investment, scaling, and incentive/policy design are necessary to bring this technology to bear, then those same commitments would be better aimed at scaling zero-emissions technologies instead. But timing is an issue. Emissions reductions are needed as quickly as possible if the world is to limit global warming to no more than 1.5°C. According to the Energy Transition Commission, for several hard-to-abate sectors with high carbon intensities, such as concrete, petrochemicals and steel, CCUS is likely to be readily available years earlier in most cases than many zero-emissions alternatives.13 In addition, the choice between CCUS and zero-emissions technologies is not binary. While true zero-emissions solutions are being scaled and deployed, more carbon-intensive processes that leverage CCUS can act as an important bridge in establishing the associated infrastructure and behavioural shifts for when those cleaner options are available. And it is here where Canada can play a significant role given an already burgeoning CCUS industry.
A prime example is in hydrogen production – one of the clean fuel sources that is expected to replace fossil fuels in the energy transition. The Hydrogen Council projects that the levelized cost of energy of zero-emissions hydrogen produced from water, or green hydrogen, could fall by as much as 58% by 2030 and will likely become cost competitive within the next 10-20 years (chart 5). However, embedding hydrogen in our energy system requires more than just cost competitiveness. A thriving hydrogen market requires significant investment in transportation infrastructure, skilled labor, and a wide array of new end-use services to be developed, including industrial and residential applications that run on hydrogen. These complementary developments are a difficult sell for energy companies, individuals and governments to invest in if the cost of the energy input doesn't reach scale until 2030 or even 2040.
However, the cost of blue or low-carbon hydrogen, produced using natural gas as feedstock and assuming high CCUS capture rates, is already at that level. In other words, using technology we have available today or relatively soon, we can notionally produce hydrogen at a level that is relatively competitive to fossil fuels and spur the additional investments in market infrastructure necessary to kickstart the transition. This creates a smoother overall transition such that when green hydrogen does reach scale, the infrastructure will already be there or in development to support it. And if CCUS capture rates reach high levels, blue hydrogen and green hydrogen can co-exist in a net zero future.
Canada is, in fact, well positioned for any market permutation of both blue and green hydrogen. The hydrogen council recently cited Canada as being a potentially competitive player in the global hydrogen space due to several factors, including ample natural gas reserves and production and the potential for renewable electricity generation to power water electrolysis for green hydrogen, including solar, wind and hydro.14 The council went on to identify several key hydrogen demand centers, including the U.S. and Europe, both of whom the International Renewable Energy Agency (IRENA) identifies as likely being net importers. Canada is already highly integrated into the North American market given the expansive network of transportation infrastructure, vertical integration of industry, and pre-existing trade relationship with the U.S. Last October, Canada also signed a Memorandum of Understanding15 with the Netherlands, with both governments agreeing to collaborate on creating a clean hydrogen export-import corridor as the latter aims to develop the Port of Rotterdam as a hydrogen distribution hub serving the rest of the European market.16
Canada is also establishing itself as a leader in CCUS technology and carbon infrastructure. The Alberta Carbon Trunk Line (ACTL), touted as the world's largest carbon transportation pipeline, currently captures approximately 2 mT of carbon emissions from an upstream bitumen upgrader and refinery (built in tandem with the pipeline) and fertilizer producer which are subsequently injected into mature oil fields for EOR. Though used exclusively for further crude oil production, the ACTL has clearly set the stage for Alberta to lead in the carbon transportation space. Three additional carbon pipelines have been proposed in the province: the TC Energy-Pembina joint Alberta Carbon Grid project, the Oil Sands Pathways to Net Zero carbon capture initiative, and the Shell Polaris project, all of which are aimed at capturing oil sands emissions and other 3rd party CO2 volumes for long-term geological storage. In addition, the Shell Quest carbon capture and storage project, jointly funded by the federal and provincial governments, has also shown significant success in capturing hydrogen production process emissions used in bitumen upgrading.17
Canada's policy landscape also sets the stage for CCUS to flourish, particularly, in the hydrogen space. The government's recent hydrogen strategy released in December 2020 outlines a clear intention to leverage the above competitive advantages and develop both blue and green hydrogen as a key fuel source, both in meeting domestic energy demand and as an economic opportunity. The government aims for hydrogen to delivering up to 30% of Canada's end-use energy demand by 2050.18
Lingering Unknowns Lie Ahead
CCUS technology development and uptake is very much still growing. Like many things in climate change scenario planning, there is a huge band of uncertainty as to how capture technology will grow and if a clear "winner" will emerge. Most agencies converge on the consensus that a combination of all available known and still-to-be-developed CCUS methods will be needed to achieve net zero emissions by 2050. This seems appropriate given specific industry carbon reduction objectives. Even within industries, individual companies will face challenges specific to their business model, the current state of operations, location, and local economy needs.
While understandable, this conclusion still leaves a complicated and somewhat unsettling feeling about the outlook of carbon capture. It also leaves a hefty plate of responsibility in the hands of companies to best assess their operations to find the capture path that suits their needs. As outlined above, the capital investment required for CCUS technologies, storage, and transportation networks is not inconsequential and estimate bands are wide as technology and efficiencies continue to evolve, making it even more difficult to plan.
This uncertainty means that the economic incentive to pursue CCUS is a key piece in scaling up carbon capture to achieve the 7.6 Gt CO2/year goal. This can come in a few forms. Taking the United States as an example, government support like the 45Q investment tax credit can simultaneously help reduce initial costs that present barriers to entry while also providing some policy certainty that might help derisk the ultimate decision to pursue this pathway. Competitive market solutions for carbon emissions, such as rising carbon prices or trading carbon offsets, can also create incentives for CCUS to become a viable short-term financial solution.
Carbon capture utilization and storage is one tool in the goal towards net zero emissions by 2050 that should not be brushed aside or eliminated. It has been tested and implemented in a variety of cases worldwide. However, much more needs to be done in scaling CCUS implementation to reach capture targets and reduce overall carbon emissions. Ultimately, companies will need to feel a financial motivation to embarking on CCUS implementation, as technology and cost uncertainties may lead to hesitation in uptake.
End Notes
- https://iea.blob.core.windows.net/assets/4719e321-6d3d-41a2-bd6b-461ad2f850a8/NetZeroby2050-ARoadmapfortheGlobalEnergySector.pdf
- https://www.ipcc.ch/site/assets/uploads/sites/2/2019/05/SR15_Chapter2_Low_Res.pdf
- https://iea.blob.core.windows.net/assets/ccdcb6b3-f6dd-4f9a-98c3-8366f4671427/The_role_of_CCUS_in_low-carbon_power_systems.pdf
- https://iea.blob.core.windows.net/assets/181b48b4-323f-454d-96fb-0bb1889d96a9/CCUS_in_clean_energy_transitions.pdf
- https://www.globalccsinstitute.com/wp-content/uploads/2021/03/Global-Status-of-CCS-Report-English.pdf
- https://netl.doe.gov/coal/carbon-capture/post-combustion
- https://www.nature.com/articles/d41586-021-02606-3
- https://www.ipcc.ch/site/assets/uploads/2018/03/srccs_wholereport-1.pdf
- https://www.energy.gov/fecm/science-innovation/carbon-capture-and-storage-research/carbon-capture-rd/pre-combustion-carbon
- https://netl.doe.gov/node/7477
- https://www.iea.org/commentaries/is-carbon-capture-too-expensive
- https://www.wri.org/insights/direct-air-capture-resource-considerations-and-costs-carbon-removal
- https://www.energy-transitions.org/wp-content/uploads/2020/08/ETC_MissionPossible_ReportSummary_English.pdf
- https://hydrogencouncil.com/wp-content/uploads/2021/02/Hydrogen-Insights-2021.pdf
- https://www.nrcan.gc.ca/climate-change/canadas-green-future/the-hydrogen-strategy/memorandum-understanding-between-the-government-canada-and-the-government-the-netherl/23907
- https://www.portofrotterdam.com/en/port-future/energy-transition/ongoing-projects/hydrogen-rotterdam
- https://www.nrcan.gc.ca/science-and-data/funding-partnerships/funding-opportunities/current-investments/shell-canada-energy-quest-project/18168
- https://www.nrcan.gc.ca/sites/www.nrcan.gc.ca/files/environment/hydrogen/NRCan_Hydrogen-Strategy-Canada-na-en-v3.pdf
Disclaimer
This report is provided by TD Economics. It is for informational and educational purposes only as of the date of writing, and may not be appropriate for other purposes. The views and opinions expressed may change at any time based on market or other conditions and may not come to pass. This material is not intended to be relied upon as investment advice or recommendations, does not constitute a solicitation to buy or sell securities and should not be considered specific legal, investment or tax advice. The report does not provide material information about the business and affairs of TD Bank Group and the members of TD Economics are not spokespersons for TD Bank Group with respect to its business and affairs. The information contained in this report has been drawn from sources believed to be reliable, but is not guaranteed to be accurate or complete. This report contains economic analysis and views, including about future economic and financial markets performance. These are based on certain assumptions and other factors, and are subject to inherent risks and uncertainties. The actual outcome may be materially different. The Toronto-Dominion Bank and its affiliates and related entities that comprise the TD Bank Group are not liable for any errors or omissions in the information, analysis or views contained in this report, or for any loss or damage suffered.
Download
Share: